Single-Particle Cryo-Electron Microscopy of Biomolecular Systems
Advances in direct electron detectors, microscope phase plates, and analysis software in the last decade have transformed single-particle cryo-electron microscopy (cryoEM) into a workhorse tool for determining near-atomic-resolution structures of large biomolecules and their complexes.
As in crystallography, the most important challenges are involved in preparing samples for measurement. The bioparticles (proteins, complexes, viruses) of interest are dissolved in buffer, deposited onto a foil supported by a grid, blotted to leave a film 10-100 nm thick, plunge cooled in liquid ethane, transferred to liquid nitrogen, and then stored, transported, and loaded into an electron microscope. With luck, the particles are uniformly and densely distributed in a monolayer, have native conformation and random orientation, the buffer in which they are embedded is fully vitrified, and the particles stay still while you take their picture in a cryo transmission electron microscope. Luck, however, is in inadequate supply, and that's where we come in.
High-resolution single-particle cryo-EM using samples vitrified in boiling nitrogen
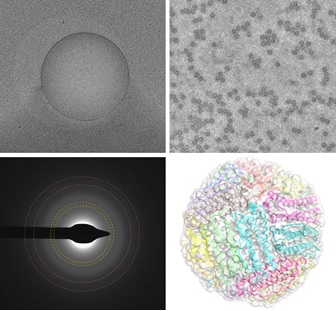
Since the Nobel-prize-winning work of Dubochet in the 1980s, cryo-EM samples have been prepared by plunge cooling in liquid ethane and then transferring them to liquid nitrogen. The use of ethane introduces complications in sample preparation protocols and in design of automated cooling instruments, and creates hazards, especially for novice users. It has been assumed (and experimentally demonstrated) that cryo-EM samples cannot be vitrified using liquid nitrogen (a less effective cryogen due to film boiling), and it has been assumed that cooling rates should be as large as possible to obtain the best imaging outcomes. Using a commercial automated cooling instrument developed at MiTeGen for cryocrystallography (based on discoveries in our Cornell group), grids and foils fabricated by MiTeGen, cryo-EM data collected at Cornell (by Katie Spoth in the CCMR) and Columbia University, and structural refinements performed by postdoc Jonathan Clinger, we showed that near atomic-resolution single-particle reconstructions can be obtained using samples cooled in boiling nitrogen. Moreover, we showed that the somewhat lower cooling rates achieved using liquid nitrogen reduce the rate of particle motion during initial irradiation - motion that blurs particle images and often limits reconstruction resolution. Drawing on ideas and results from my Cornell research, we gave detailed explanations for these results, and for why they differed from those obtained by Dubochet and others.
High-resolution single-particle cryo-EM of samples vitrified in boiling nitrogen. T. Engstrom, J. A. Clinger, K. A. Spoth, O. B. Clarke, D. S. Closs, R. Jayne, B. A. Apker and R. E. Thorne, IUCrJ 8, 867-877 (2021).
Origin of electron beam-induced motion during imaging

When a sample comprised of biomolecular particles in a thin ice film is imaged in an electron microscope, the particles don't stay still but instead move during electron irradiation, blurring the images. Motion is fastest at the start of irradiation, when the particles have suffered the least amount of radiation damage, and where the information content of the imags would otherwise be highest. This electron-beam-induced motion prevented high resolution reconstructions until the development of efficient, high-frame-rate direct electron detectors. These allow "movies" of particle motion to be acquired, which can be processed to correct for motion. However, motion correction is imperfect, and the mechanism of this beam induced motion (BIM) has been unknown.
We proposed that BIM is due to electron-irradiation-induced creep in the sample film, driven by primarily compressive stresses within the film. These stresses arise primarily from transient differences in temperature as the grid and foil cool at different rates toward their final temperature. The particle-containing sample vitrifies on a tensile-stressed foil, and when this tensile stress is released the sample film is left in compressive stress. Electron irradiation in the presence of this compressive stress drives observed doming motion of the sample film within each foil hole. This mechanism is consistent with all available data for BIM.
Hypothesis for a mechanism of beam-induced motion in cryo-electron microscopy. R. E. Thorne. IUCrJ 7, 416-421 (2020).