Physics of Cryopreservation
Cooling samples to low temperatures is important not only in protein structure determination, but in the long-term preservation of proteins, viruses, cells and tissues. The basic methods used by biological, medical and veterinary practitioners to freeze and store biological samples have remained largely unchanged for at least two decades, and were arrived at empirically rather than through systematic studies based on understanding of fundamental principles. We are studying both fundamental and applied problems in cryopreservation.
Ice nucleation and critical cooling rates
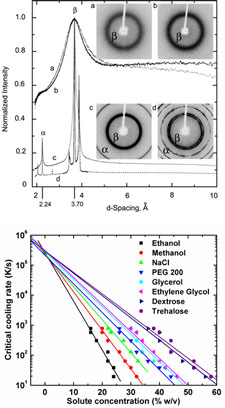
Previous studies of ice formation have focused on nucleation and growth at cooling rates of 0.01 to 10 K per second. Using X-ray, optical, and thermal measurements, we have developed methods for determining the minimum or critical cooling rates required to obtain ice-free, vitrified low-temperature states for cooling rates up to 100,000 K per second. This allows study of nucleation and vitrification at much lower solute concentrations and thus over a much wider range of solute concentrations.
We have used these methods to measure critical cooling rates for more than a dozen different solutes, including most of those commonly used as cryoprotective agents. For all solutes studied, the critical cooling rate varies exponentially with solute concentration, and the zero concentration extrapolation matches the measured critical cooling rate for pure water.
Effects of cryoprotectant concentration and cooling rate on vitrification of aqueous solutions. V. Berejnov, N. S. Husseini, O. A., Alsaied and R . E. Thorne, J. Appl. Cryst. 39, 244-251 (2006).
Hyperquenching for Protein Crystallography. M. Warkentin, V. Berejnov, and R. E. Thorne, J. Appl. Cryst. J. Appl. Cryst. 39, 805-811 (2006).
Cryocrystallography in capillaries: critical glycerol concentrations and cooling rates. M. Warkentin, V. Stanislavskaia, K. Hammes and R. E. Thorne, J. Appl. Cryst. 41, 791-797 (2008).
Theory of glass formation in the presence of solutes
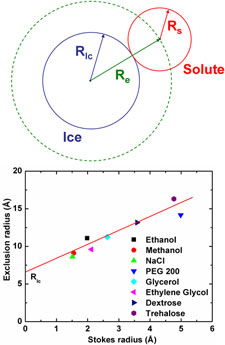
To explain our critical cooling rate data, we have developed a new theory of glass formability in aqueous solutions, using statistical mechanics to extend classical nucleation theory and account for the effects of solutes. We argue that solute molecules must be excluded from the critical nucleus, so that the barrier to ice nucleation is increased by the solute's osmotic pressure. This results in an exponential reduction in nucleation rate - and thus of the critical cooling rate - with solute concentration, and the observed . Using this theory, we have extracted effective interaction radii for each cryoprotectant from critical cooling rate data. These radii vary linearly with the cryoprotectant's hydrodynamically determined Stokes radius, consistent with our theory.
Critical droplet theory explains the glass formability of aqueous solutions. M. Warkentin, J. P. Sethna and R. E. Thorne. Phys. Rev. Lett. 110, 015703 (2013).
Critical warming rates, devitrification and recrystallization
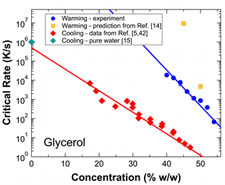
Ice formation on warming is of comparable or greater importance to ice formation on cooling in determining survival of cryopreserved samples. We have determined critical warming rates required for ice-free warming of vitrified aqueous solutions of several common cryoprotectants, at warming rates of order 10 to 10,000 K/s. Critical warming rates are typically one to three orders of magnitude larger than critical cooling rates, and vary strongly with cooling rate. Current models with current best estimates for input parameters provide a reasonable account of critical warming rates at high concentrations/low rates, but overestimate both critical warming and cooling rates by orders of magnitude at lower concentrations and larger rates.
Effect of common cryoprotectants on critical warming rates and ice formation in aqueous solutions. J. B. Hopkins, R. Badeau, M. Warkentin and R. E. Thorne, Cryobiology 65, 169-178 (2012).
Density of aqueous glasses at cryogenic temperatures
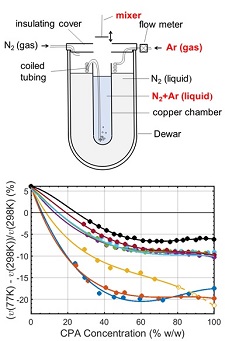
Protein crystals and cells are typically soaked in aqueous solutions containing between 15% and 60% w/v of cryoprotectants such as glycerol, ethylene glycol, or sucrose. Contraction of these solutions on cooling exerts stresses on the crystals and cells that may damage them. We have made the first measurements of the cryogenic-temperature densities of several aqueous cryoprotectant solutions at concentrations below 50% w/w using a novel buoyancy-based method, and used these to determine the volume contraction of these solutions on cooling to cryogenic temperature.
Thermal contraction of aqueous glycerol and ethylene glycol solutions for optimized protein crystal cryoprotection. C. Shen, E. F. Julius, T. J. Tyree, D. W. Moreau, H. Atakisi, and R. E. Thorne. Acta Cryst. D 72, 742-752 (2016).
Measuring the densities of aqueous glasses at cryogenic temperatures. C. Shen, E. F. Julius, T. J. Tyree, R. Dan, D. W. Moreau, and R. E. Thorne, JoVE 124, e55761 (2017).
Density and electron density of aqueous cryoprotectant solutions at cryogenic temperatures for optimized cryoprotection and diffraction contrast. T. J. Tyree, R. Dan, and R. E. Thorne. Acta Cryst. D 74, 471-479 (2018).
Ultra-fast cooling
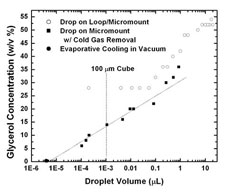
The fastest cooling rates are obtained by plunging samples from air into a cryogenic liquid such as nitrogen, propane or ethane. We have demonstrated a simple plunge cooling method that increases cooling rates for small samples by a factor of 20-100 over previous best practice. These cooling rates - 20,000 to 100,000 K/s - allow even protein-free aqueous solutions to be vitrified with very small (<10%) cryoprotectant concentrations.
Above any cold liquid surface there will be a layer of cold gas, formed by conduction and convection and, if the cryogenic liquid is near its boiling point, by evaporation. For sufficiently small samples, most cooling occurs in this gas layer, not in the liquid, at a rate determined by heat transfer to the gas. Our measurements showed that "sufficiently small" is smaller than roughly 500 microns - which is larger than nearly all protein crystals. By simply blowing this cold gas layer away immediately prior to the sample plunge, we increased cooling rates from ~1000 K/s to ~20,000 K/s for ordinary size crystals.
Hyperquenching for Protein Crystallography. M. Warkentin, V. Berejnov, and R. E. Thorne, J. Appl. Cryst. J. Appl. Cryst. 39, 805-811 (2006).